Soil is not just a layer of earth beneath our feet, but a vibrant hub of life, a nexus where countless organisms coalesce in complex networks of existence. Did you know that, in just a handful of healthy soil, there are more living organisms than there are people on our planet? These microscopic custodians play a pivotal role in nutrient cycling, soil fertility enhancement, and organic matter decomposition, thereby creating a symbiotic relationship with plants to cultivate thriving microbial ecosystems that help to sustain world nutrition. Accordingly, the future of sustainable development and biodiversity conservation in agriculture requires a thorough understanding of the profound importance of soil in orchestrating plant-microbe interactions and sustaining ecological balance.
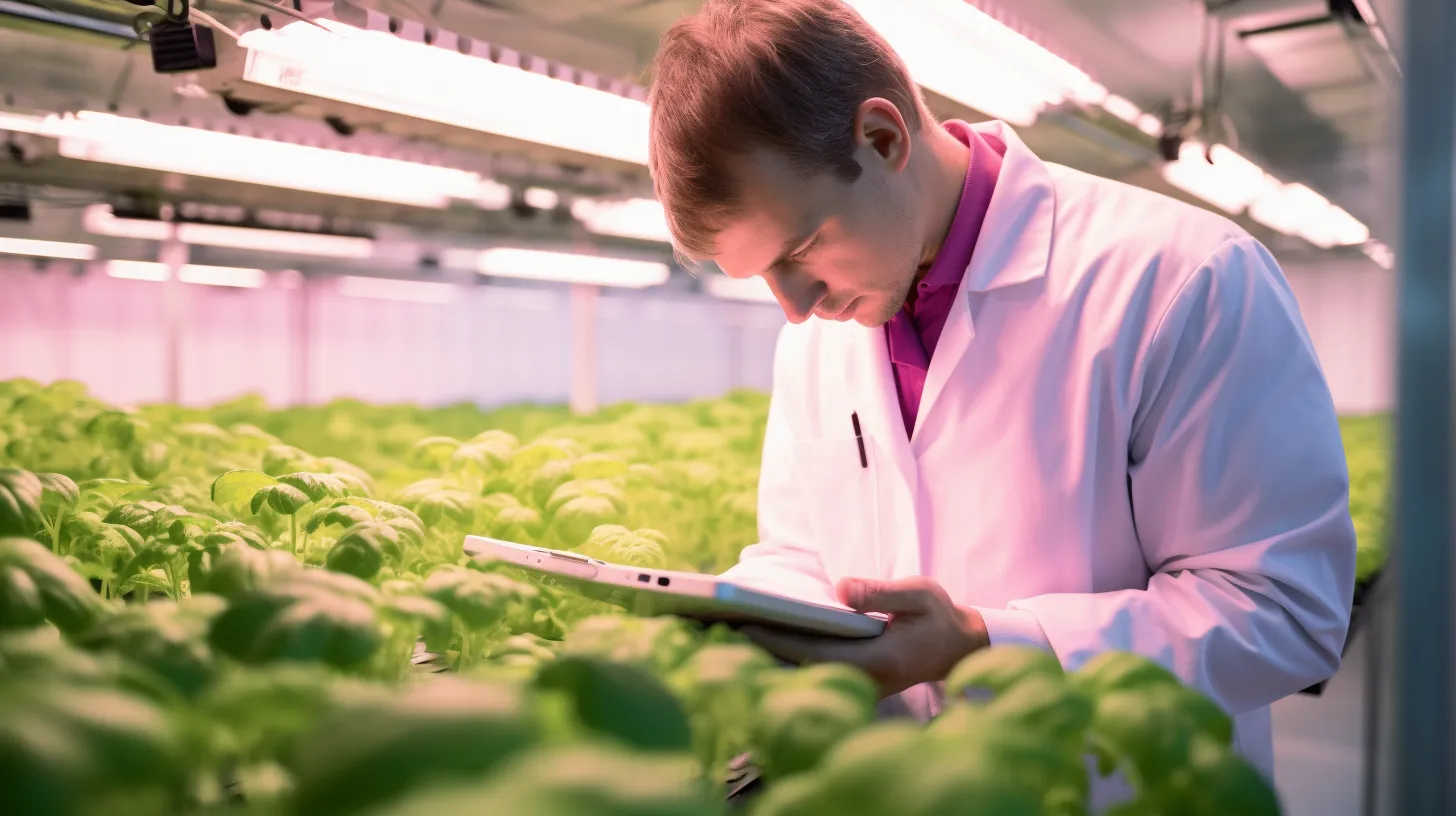
Understanding Soil Metagenomics
Soil metagenomics is a leading area of soil research that explores soil microorganisms using genetic analysis. Soil metagenomics helps us to better understand soil biology and its critical functions in the ecosystem. In view of the importance of soil and its microorganisms, in this article, we discuss how soil metagenomics advances soil biology and supports regenerative, sustainable agriculture.
Metagenomics and Its Application in Studying Soil
Metagenomics is an advanced microbiology approach that studies entire microbial communities directly from environmental samples like soil, i.e. without culturing individual microorganisms. Analyzing soil DNA’s genetic material makes it possible to identify microorganisms’ genomes in a specific ecosystem. This revolutionary approach provides a comprehensive understanding of soil microbiomes and their roles in nutrient cycling, organic matter breakdown, and overall soil health.
How Metagenomics Differs From Traditional Soil Testing Methods
Metagenomics, which studies soil microorganisms’ genetic material directly from environmental samples, regardless of sample type or the number of microorganisms in it, is a relatively new method in soil research. Overcoming the inherent limitation of traditional soil research methods that, due to their focus on culturing specific microorganisms, frequently overlook many others, metagenomics directly analyzes soil genetic material using DNA sequencing technology. Using metagenomics, researchers can uncover a wide array of microorganisms and identify functional genes responsible for vital ecological processes. As a powerful tool for studying soil microbial communities, the metagenomic approach provides a granular account of the microbial composition of a soil sample or potential functions of the microbes inhabiting it, thereby opening new doors to understanding previously unculturable microorganisms and their role in the soil ecosystem.
Key Components of Soil Metagenomics
Soil is an interconnected self-organized system with high levels of exchange of energy between organisms and physicochemical components. Soil metagenomic analysis unfolds in several stages, each providing valuable information about how microorganisms interact with each other in soils. In what follows, each of these stages is described in further detail.
1. Sample Collection
The first step in soil metagenomics is collecting representative soil samples from different locations or environments. Here, careful sampling techniques ensure that capturing a wide range of microorganisms for subsequent analysis.
2. DNA Extraction and Sequencing
The second step is extracting DNA from the soil samples, with a particular focus on rhizosphere, to obtain genetic material of the microorganisms from the soil and plant. To read and analyze this genetic information from the soil, RhizeBio’s advanced soil DNA sequencing technology is used.
3. Bioinformatics Analysis
The third step in soil metagenomics is processing and analyzing the sequenced soil data using specific bioinformatics tools and algorithms, such as quality control, assembling short reads into longer sequences, classifying sequences by taxonomy, interpreting their functions, and conducting comparative analysis.
4. Data Interpretation
Finally, the fourth step is data interpretation. This includes identifying specific microbes present in the sample, assessing their functional potential based on specific genes, and exploring the relationships between microbial communities and environmental factors.
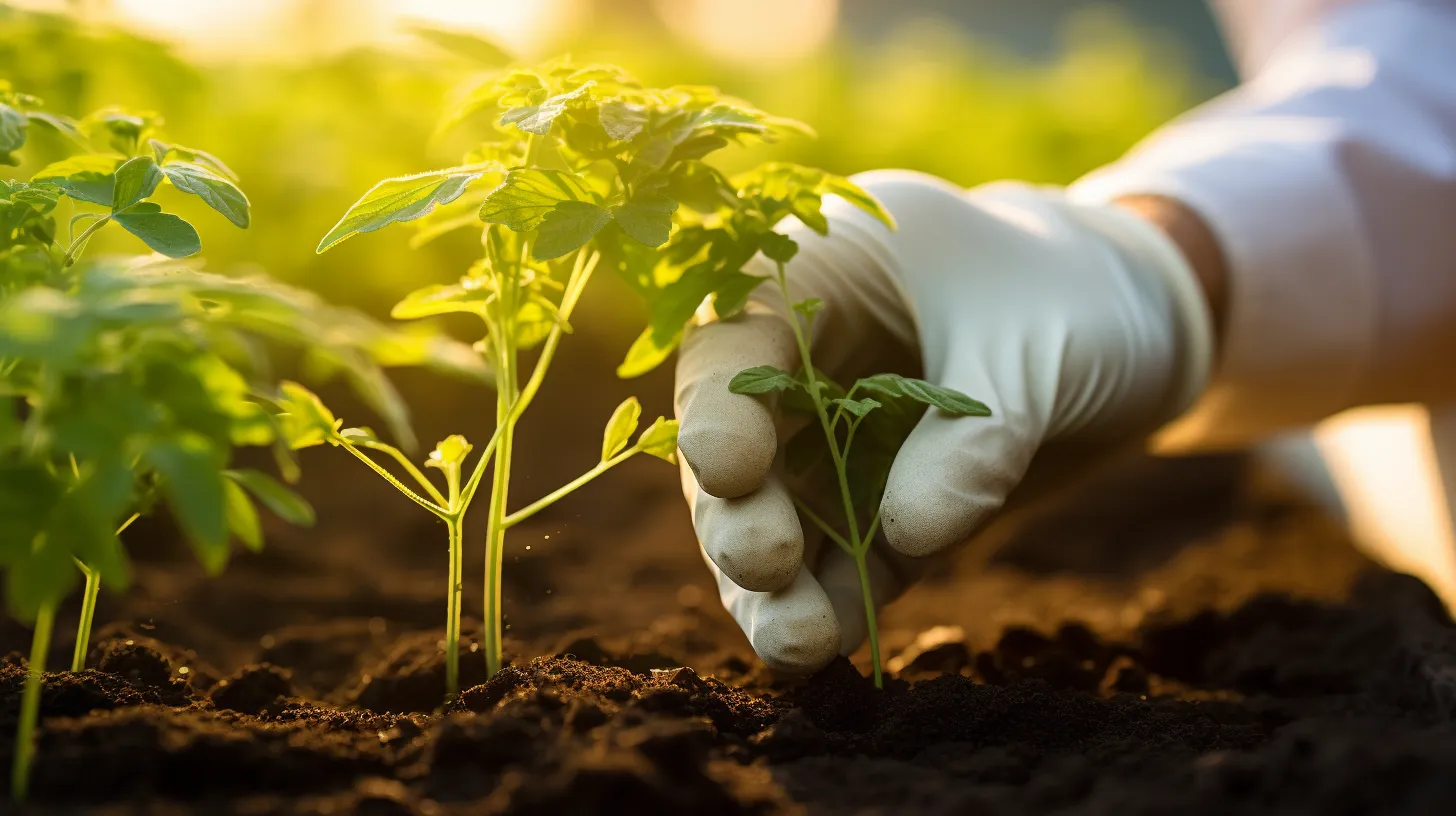
The Hidden World of Soil Microbes
Microorganisms Present in Soil
Soil microorganisms play a pivotal role in supporting biodiversity and nutrient recycling. Accordingly, research on these microorganisms can help to inform agricultural practices that promote sustainable practices while preserving soil biodiversity. The following types of microorganisms that contribute to the balance and productivity of the soil ecosystem can be discerned:
Bacteria – Bacteria in the soil help cycle nutrients, break down organic matter, and fix nitrogen, which provides nutrients to plants in the soil ecosystem.
Archaea – Capable of surviving in extreme conditions, archaea contribute to nutrient cycling processes such as nitrification and denitrification.
Fungi – Through forming mycorrhizal associations with plant roots, fungi improve nutrient uptake, decompose organic matter, and release nutrients.
Virus – Via infecting other microorganisms, viruses control microbial diversity and populations.
Protozoa – Protozoa, which regulate microbial populations, impact nutrient cycling.
Algae – Contributing to nutrient cycling and water retention, algae support higher organisms.
Challenges of Studying Soil Microbes with Traditional Methods
Studying soil microbes using traditional methods is associated with several challenges, such as difficulty in culturing many soil microorganisms, time-consuming procedures, and limitations in capturing comprehensive interactions among soil microbes.
Traditional methods may capture only a fraction of the diverse microbial community. Another limitation of traditional methods is their reliance on culturing the microbes in the laboratory, which can be very labor-intensive and slow. Finally, focusing on isolated individual soil microbes and their individual properties does not provide a comprehensive picture of the complexity of functions performed by entire microbial communities.
The limitations of traditional methods briefly reviewed above highlight the significance of using advanced and comprehensive approaches like metagenomics.
Advantages of Soil Metagenomics
A powerful tool for studying genetic material in soil samples, soil metagenomics makes it possible to obtain a comprehensive view of microbial communities, discover novel genes and metabolic pathways, and get insights into functional gene capabilities, all of which offer meaningful implications for sustainable agriculture and environmental management. Analyzing DNA from diverse soil microorganisms can help to both extend the current theoretical knowledge about soil ecosystems and obtain valuable information with potential practical applications in various fields.
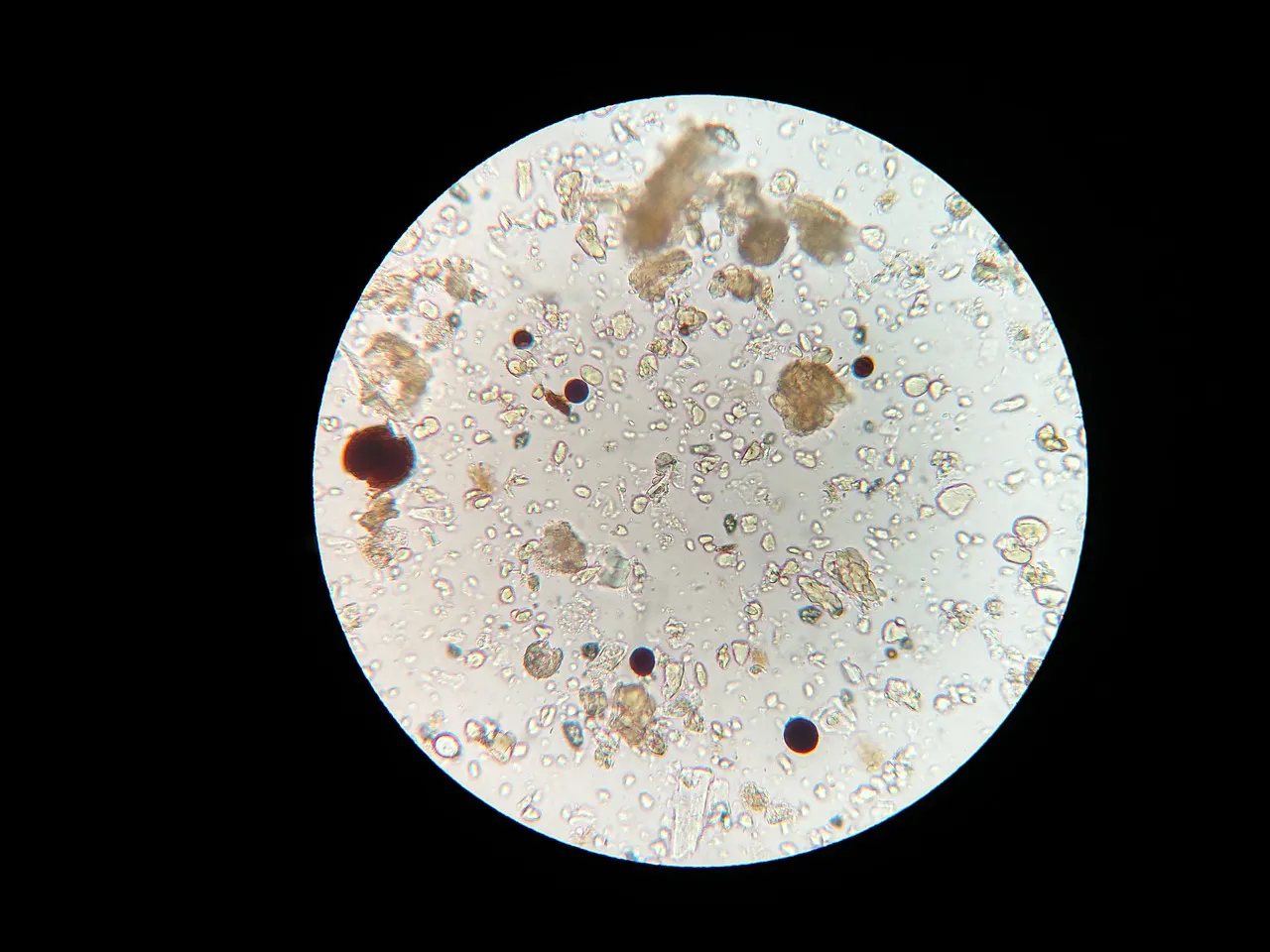
Understanding the Complexity of Soil Microbial Communities
Complexity of soil microbial communities stems from the variety of species and intricate interactions among them. Different species have unique roles, ensuring efficient nutrient cycling, organic matter decomposition, and disease suppression. Soil microbial communities are influenced by a broad range of environmental factors, such as temperature and land management, which cause dynamic changes and diversity over time.
The complexity of soil microbial communities arises from the following factors:
-
Diversity
Soil microbes are incredibly diverse, with billions of microorganisms per gram of soil. This diversity arises from varying environmental conditions and available organic matter.
-
Spatial Heterogeneity
Microbes are unevenly distributed in soil. Depending on soil properties, plant presence, and site history, patches with different compositions are formed.
-
Interactions
Microbes interact with each other and the environment, impacting community functions. Some produce antibiotics, while others form beneficial relationships with plants.
-
Dynamics
Under the impact of environmental shifts, introduction of new microbes, and natural mortality, soil microbial communities are dynamic and constantly evolve.
Discovery of Novel Microorganisms and Functional Genes
Identifying novel microorganisms and functional genes—i.e., those that have not been previously described or cultured—refers to finding new microbes and their specific functions in an ecosystem.
On the one hand, identification of novel microorganisms expands our understanding of microbial diversity and ecological roles of microorganisms. As demonstrated by Handelsman et al. (1998), soil microorganisms are essential sources of novel natural compounds.
On the other hand, identifying functional genes—i.e. specific genes responsible for performing essential functions, such as nutrient cycling, organic matter decomposition, and disease resistance—sheds light on the potential roles of different microorganisms in the ecosystem and their contributions to key processes.
The discovery and characterization of novel microorganisms and functional genes contribute to advancing our understanding of microbial ecology and the corresponding interactions in ecosystems. This information may offer important implications for agriculture, biotechnology, and environmental conservation, thereby creating opportunities for sustainable practices and innovative applications in different industries.
Assessing The Impact of Environmental Factors on Soil Microbial Diversity
Soil microbes’ interaction with environmental factors affects the composition of microorganisms in the soil. Among the many environmental factors that can influence soil microbial diversity are the following:
Temperature – Soil temperature may have a significant impact on soil microbial composition. The rule of thumb here is that, with an increase of temperature, microbial activity also increases.
pH Level – Although most soil microorganisms prefer a neutral soil pH, some microorganisms can tolerate extremely high pH values.
Moisture – Most soil microbes need moisture to survive. However, too much moisture can also be harmful to soil microbes.
Nutrient Availability – Most soil microbes need a variety of nutrients to survive, which has a direct impact on soil microbial diversity.
Soil Type – Since chemical and physical properties of different soil types vary, each soil type is supportive of a specific range of microorganisms.
Pollution – Certain pollutants can harm or kill soil microbes, and some can disturb their interactions, which has a negative impact on microbial diversity.
With its advanced, next-generation DNA sequencing, RhizeBio provides farmers with efficient soil testing services to evaluate the intrinsic capabilities of their soil’s microbes, ensure an efficient cycling of nutrients, and combat diseases on the farm.
Applications of Soil Metagenomics
1. Enhancing Soil Fertility and Nutrient Cycling
Soil metagenomics has dramatically changed the way we look at soil biology and its applications in plant growth and nutrient cycling. One key application of soil metagenomics is enhancing soil fertility by identifying the genes involved in nutrient acquisition and cycling, which results in the introduction of more targeted strategies such as microbial inoculants or biofertilizers.
Additionally, soil metagenomics helps to assess microbial diversity and community structure, informing soil health and nutrient cycling management. Soil metagenomics facilitates comprehending the effects of diverse sustainable farming practices on the soil microbiome and encourages the adoption of practices that enhance the resilience of the soil microbiome. This could result in higher crop yields and greater sustainability.
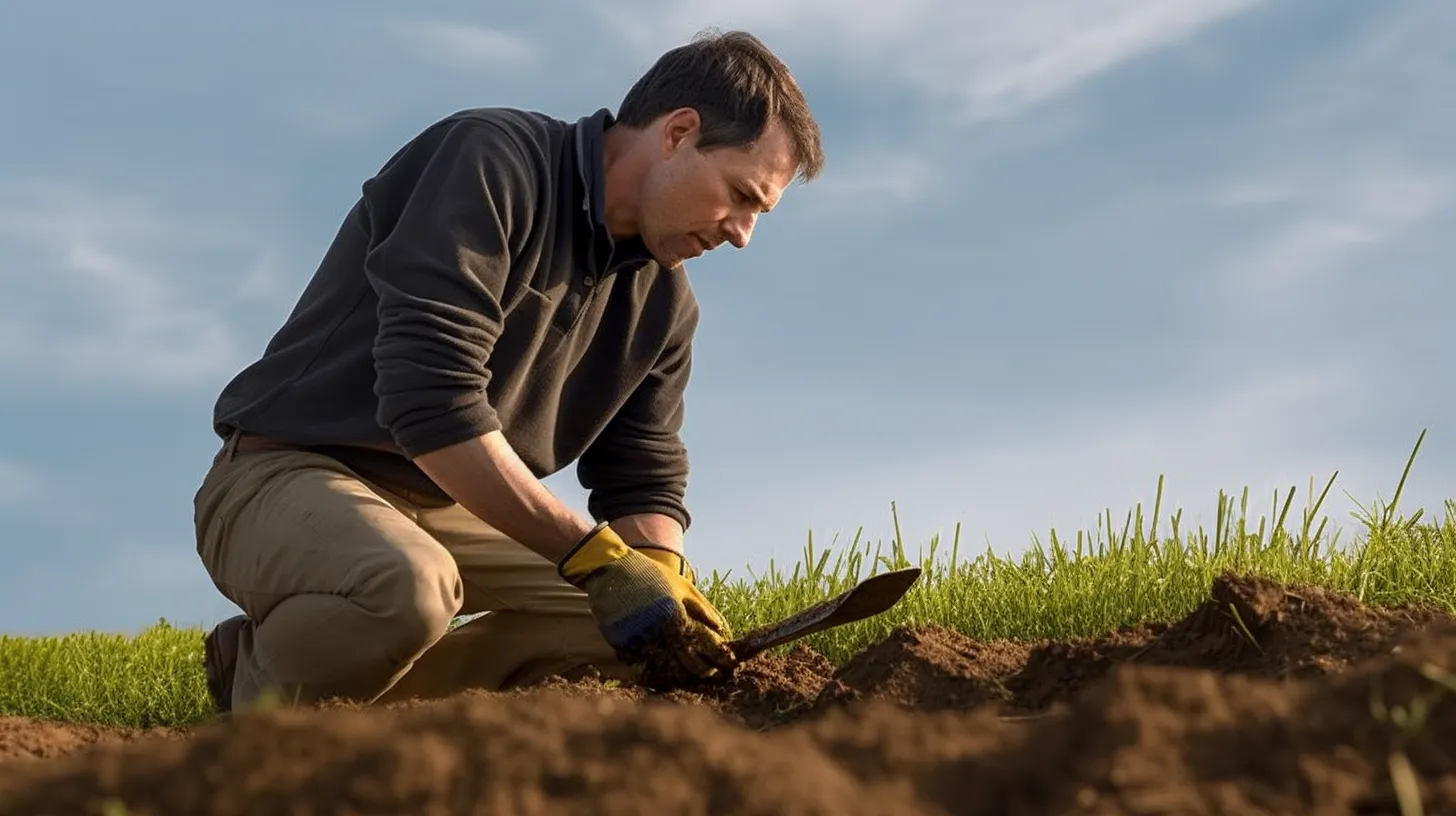
2. Bioremediation and Soil Pollution Management
Another important application of soil metagenomics is bioremediation. In bioremediation, through an analysis of genetic diversity and related genes, the soil metagenomic approach is used to identify pollutant-degrading microorganisms. This promotes the use of targeted strategies, such as introducing specific microbial groups or enhancing the existing ones. It also tracks how microbial communities respond to pollution, guiding effective management.
Moreover, soil metagenomics enables uncovering new enzymes and metabolic pathways for eco-friendly soil pollution solutions, as well as offers abundant possibilities in sustainable environmental management, from identifying pollutant-degrading microorganisms to monitoring ecosystem responses and discovering novel pathways. All this makes soil metagenomics a vital asset for a greener future.
3. Contribution to Sustainable Agriculture and Plant Health
Soil metagenomics also contributes to sustainable agriculture and plant health. Studying soil’s genetic material to gain insights into crucial microbial communities affecting soil fertility, nutrient cycling, and disease suppression, soil metagenomics makes agriculture more sustainable, as well as informs farmers on crop rotation, organic fertilization, and pest management. Along with optimizing resource use and minimizing environmental impact, it also improves plant health by identifying beneficial microorganisms that enhance growth and fight pathogens, thereby promoting the development of eco-friendly biofertilizers and biopesticides. Furthermore, soil metagenomics enhances crop resilience in challenging conditions such as drought or salinity, promising sustainable agriculture and resilient crops in the face of climate change.
Finally, in full alignment with current social and environmental policies, sustainable agricultural practices promoted by soil metagenomics help to achieve a better balance between agricultural productivity and environmental protection, aligning it with social and environmental policies.
Soil Metagenomics Techniques and Challenges
Key soil metagenomics techniques include DNA extraction and sequencing. Data analysis, gene identification, and microbial genome reconstruction are performed using bioinformatics tools such as MG-RAST, QIIME, and MEGAN.
However, despite recent advances in soil metagenomics, the use of metagenomic approaches is associated with certain challenges and limitations. First, soil heterogeneity and inhibitors can adversely impact DNA extraction and sequencing accuracy. Second, linking genetic information to specific microorganisms or traits remains complex. Yet overcoming these obstacles and advancing experimental and computational techniques will deepen our understanding of soil ecosystems and their vital role in the environment.
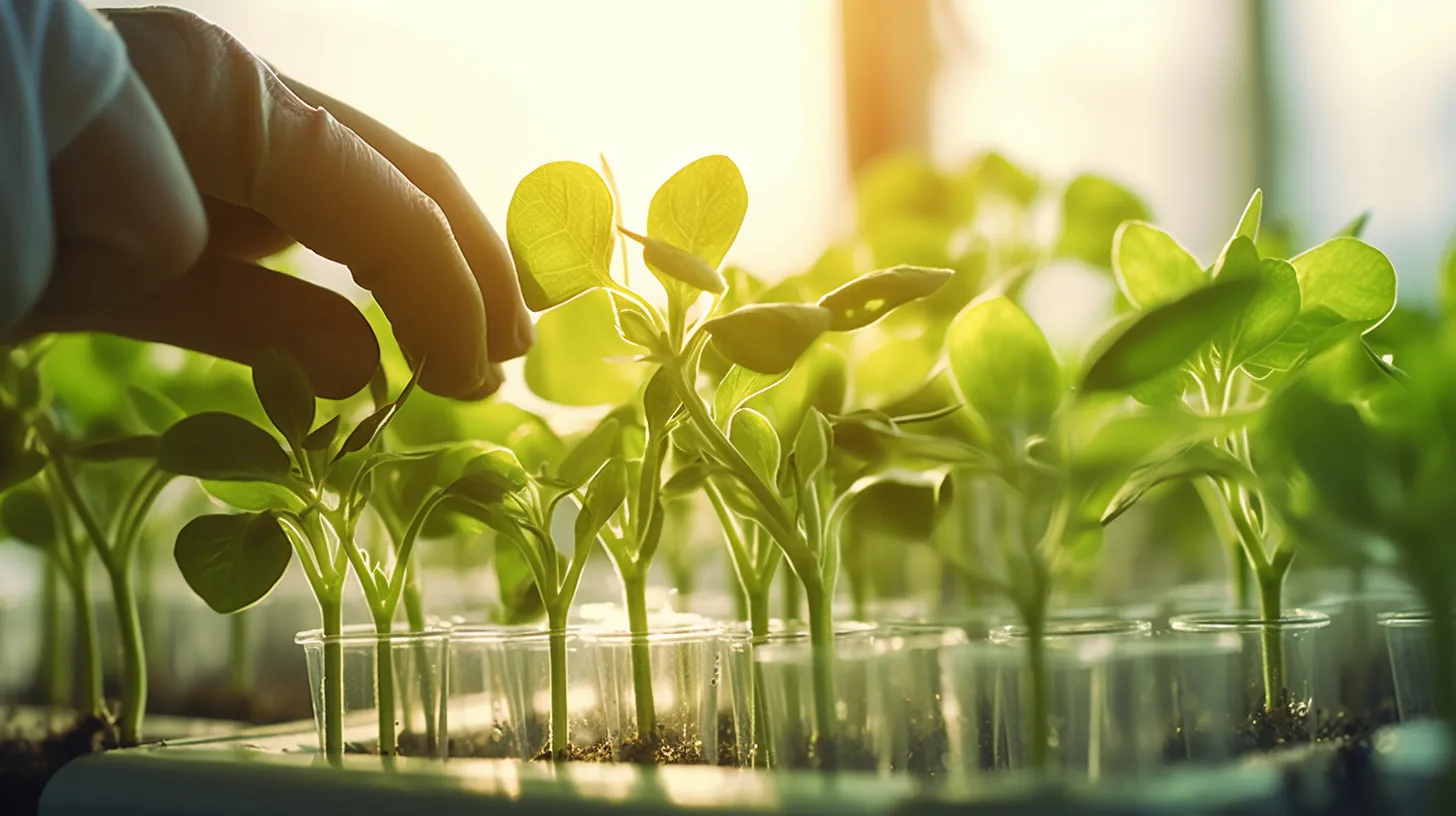
Case Studies: Soil Metagenomics in Action
1. Understanding Soil Microbiology using DNA Sequencing
-
Study
A research team conducted a soil metagenomics investigation of agricultural fields with contrasting management practices, including conventional, organic, and regenerative systems. The researchers analyzed the soil DNA to characterize the microbial communities and evaluated their functional potential.
-
Outcomes and Implications
The results of this study revealed distinct microbial compositions and functional profiles in each management system. Organic and regenerative fields exhibited a higher microbial diversity and an abundance of beneficial microbes associated with nutrient cycling and disease suppression. In previous research, metagenomics was used to detect microbiome genomic characteristics involved in plant-microbial interactions (Bulgarelli et al., 2015; Xu et al., 2018). By contrast, conventional fields had lower diversity and an increase in potentially harmful pathogenic species. These findings highlight the importance of sustainable agriculture practices in fostering healthy soil microbial communities and their potential benefits for crop health and yield.
-
Broader Applications
The results of this study clearly demonstrate the potential for soil metagenomics to guide agricultural practices towards more sustainable and regenerative practice. Some microorganisms associated with plants in soil were also reported to have characteristics beneficial for plant growth (Sessitsch et al., 2012; Xu et al., 2018; Carrión et al., 2019; Li et al., 2019). Therefore, taking into account microbial indicators of soil health identified by soil metagenomic analysis, farmers can improve nutrient cycling, minimize chemical inputs, and boost soil fertility. This valuable knowledge opens doors for developing customized microbial inoculants and biofertilizers, catering to specific crops and regions, and fostering eco-friendly and economically viable farming techniques.
2. Assessing Soil Contamination using Metagenomics
-
Study
In another real-life application of soil metagenomics, a team of environmental scientists evaluated the extent of soil contamination in an industrial area. By analyzing the genetic material in soil samples, the researchers were able to identify and characterize the microbial communities residing in contaminated sites.
-
Outcomes and Implications
The findings demonstrated the effectiveness of the soil metagenomic approach for assessing environmental pollution. By comparing the microbial diversity and abundance in contaminated and uncontaminated soils, the researchers gained valuable insights into the impact of pollutants on soil ecosystems. This information can aid in developing effective strategies for soil remediation and pollution management.
-
Broader Applications
This study demonstrated the potential for broader applications of soil metagenomics across various industries. Going beyond environmental monitoring, metagenomic analysis finds applications in a wide range of fields, including but not limited to pharmaceutical research, waste management, and bioremediation. Quick identification and characterization of microbial communities enable creating novel enzymes, antibiotics, and bioactive compounds.
These real-life examples highlight the advantages of using soil metagenomics in revealing microbial diversity, evaluating soil pollution, and inspiring innovation in different sectors. Metagenomic analysis provides deeper insights into the soil microbiome, thereby offering novel opportunities for sustainable agriculture, environmental care, and biotechnology progress.
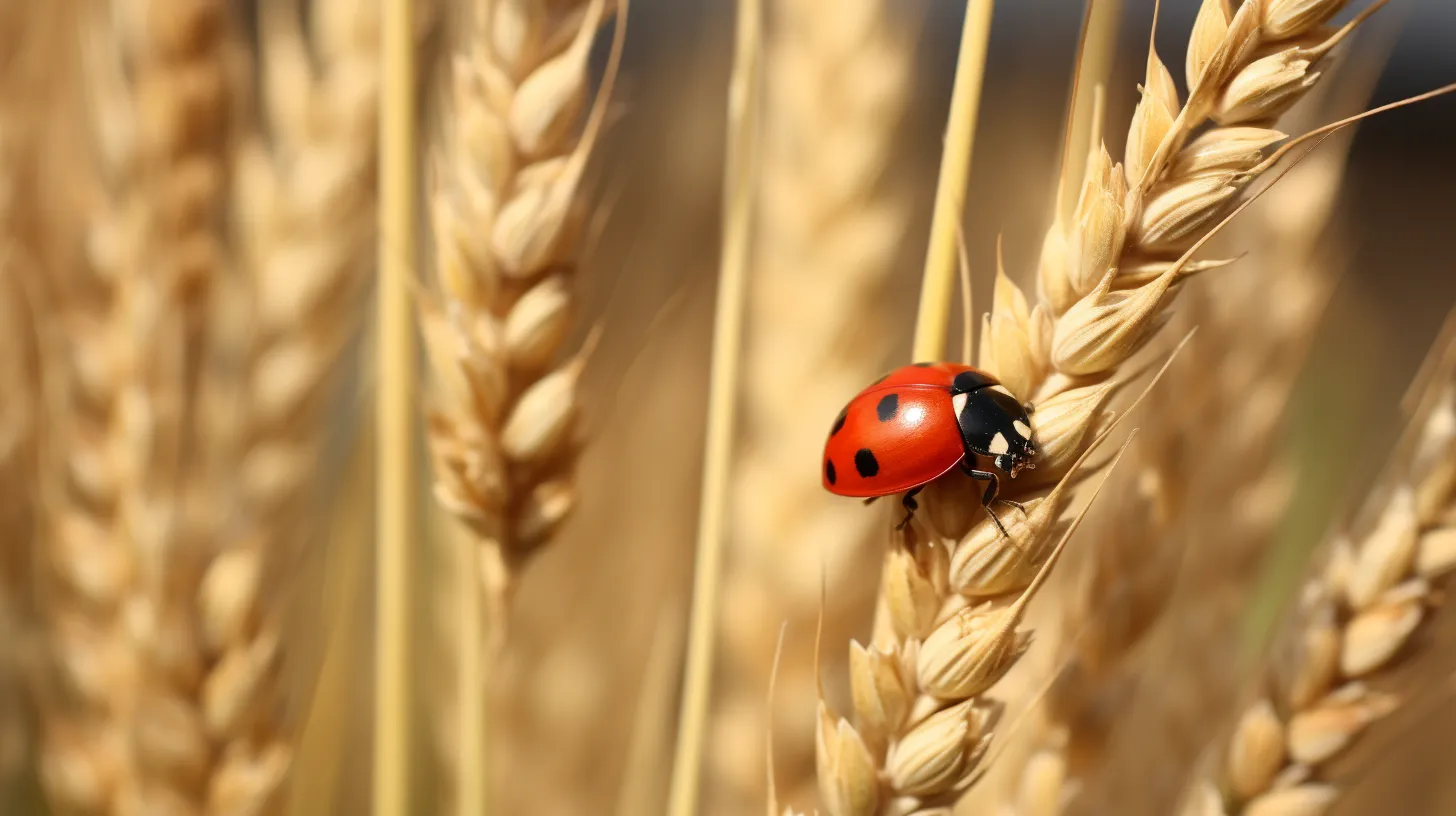
Best Practices for Conducting Soil Metagenomics Studies
Proper sampling techniques and considerations
Effective soil metagenomics begins with proper sampling techniques that enable capturing diverse soil microorganisms. Here, a crucial aspect is selecting appropriate sampling locations representative of the diversity of a specific soil ecosystem. Researchers should employ random sampling methods and prioritize contamination prevention using sterilized tools and protective equipment. Comprehensive data collection can also be ensured by taking into account aspects such as soil type, land use, and environmental conditions. These considerations form the basis for accurate and insightful soil metagenomics studies.
Standardized protocols for DNA extraction and sequencing
Standardized protocols for DNA extraction and sequencing help to prevent technical biases that could adversely affect the data. In addition, standardized protocols ensure consistency across studies, facilitate comparisons between research groups, and minimize variations. Standardized sequencing protocols also reduce technical discrepancies and enhance reproducibility. Confident data interpretation without contamination or bias sources also requires the implementation of best practices such as quality control measures. With the advanced soil DNA sequencing technology, RhizeBio’s soil DNA diagnostic tools stand out as a game-changer and prove to be more valuable to farmers than other soil testing methods.
Effective interpretation and validation of results
Effective interpretation and validation of the results is essential in soil metagenomic research to ensure reliability and accuracy of the collected data. In terms of data interpretation, a useful approach is comparing the results with reference database(s), which helps to identify microbial composition trends.
Equally important is validation of the results, which involves techniques like statistical analysis, replication, and cross-validation. Specifically, statistical analysis gauges the significance of differences, while replication ensures consistency across experiments. Cross-validation confirms results by comparing different methodologies. The use of these validation methods ensures accurate findings. Accordingly, adoption of these practices is the best way to draw meaningful and accurate conclusions from soil metagenomic studies.
Overcoming Challenges and Future Prospects
Addressing data complexity and analysis challenges
The soil metagenomic analysis of complex datasets is associated with certain challenges. First, the diverse genes in soil microorganisms require the use of advanced bioinformatics tools. Furthermore, metagenomic analysis requires a deep understanding of math models to make sense of sequencing data and find patterns in genomics and soil ecology. Last but not least, accuracy of the results is challenged by differences in microorganisms caused by the environment.
Despite these difficulties, soil metagenomics has a bright future. New sequencing methods make studying genes affordable. Better computer methods, such as machine learning, provide a more comprehensive account of the data. Finally, using advanced tools and tech helps researchers to learn more about the soil world.
Future directions and emerging technologies in soil metagenomics
The future of soil genomics depends on better metagenomic technologies. As gene-reading methods get cheaper and easier to use, soil metagenomics research will develop in close interaction with other areas of research, such as multi-omics approaches, metatranscriptomics, metabolomics, and proteomics.
Future advances in soil genomics are also intrinsically linked to the development of computer tools and machine learning techniques that will help us to learn more about keeping soil healthy.
Potential impact on agriculture, environmental conservation, and human health
We uncover the roles and functions of microorganisms through the genetic analysis of soil samples.
In agriculture, soil DNA testing is used to identify beneficial microbes for plant growth and disease resistance. This knowledge promotes eco-friendly farming and helps agronomists to make informed decisions for their farms. Additionally, from metagenomic findings, environmental conservation can gain insight into carbon cycling and pollutant breakdown, which aids soil restoration and climate action.
With regard to its impact on human health, soil metagenomics is instrumental in identifying microbial impacts on food quality and potential antimicrobial compounds. Furthermore, soil metagenomics is also instrumental in discovering helpful microbes—for instance, those capable of producing useful substances—that can be used in medicine and technology fields for various purposes. RhizeBio’s technology plays a major role in this area of discovery.
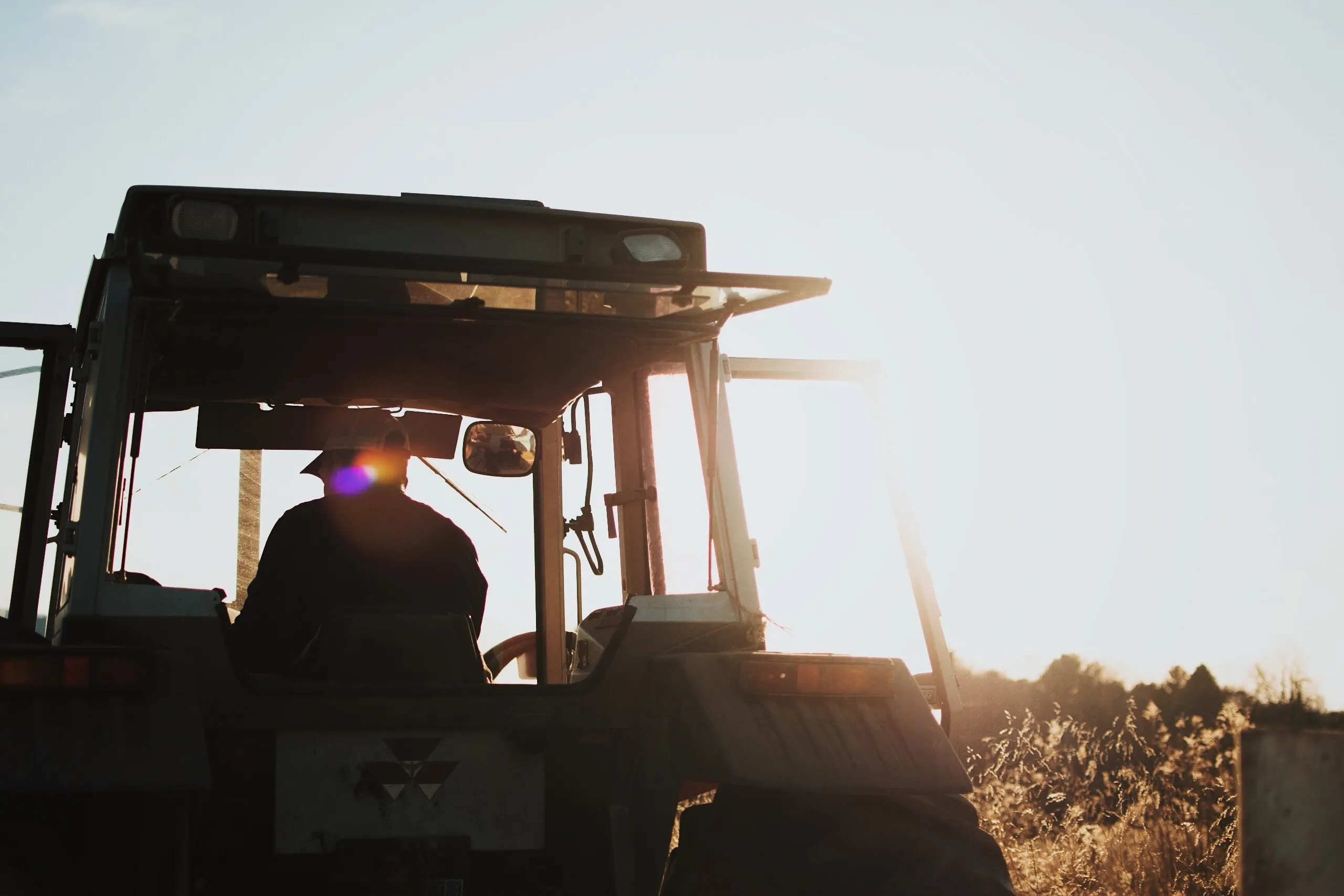
Conclusion
In summary, soil metagenomics is a groundbreaking approach that advances our understanding of soil biology through genetic analysis of soil samples and offers valuable insights into diverse microbial communities. The results of soil metagenomic analysis can clarify intricate relationships among soil microorganisms that affect nutrient cycling, plant health, and fertility. The knowledge afforded by soil metagenomics supports sustainable agriculture via optimizing crop yields while minimizing adverse environmental impacts. In addition, soil metagenomics can be meaningfully used to identify new microbial species and functions, with potential biotechnological applications.
Using metagenomic approaches, researchers can further explore the genetic makeup of soil microorganisms and derive valuable insights into their roles and interactions. Based on the findings, more informed decisions in sustainable agriculture, environmental conservation, and human health can be made. Through collaboration among researchers, industries, and policymakers, soil metagenomics can be effectively integrated into practices and policies that enhance soil health and ecosystem preservation, thereby empowering productive and environmentally friendly practices while fostering a resilient and sustainable ecosystem.
Considering these benefits, and in view of the promising potential of soil metagenomics in revolutionizing soil biology research, offering solutions to global challenges in food security and environmental sustainability, and shaping a resilient future, it should be adopted as a valuable tool to safeguard ecosystems for the future.